Determining the preeminent plastic wastes in the production of petrol using pyrolysis method and its effectiveness as an alternative fuel
DOI:
https://doi.org/10.29303/aca.v7i1.189Keywords:
Polyethylene Terephthalate (PET), High-Density Polyethylene (HDPE)l., Low-Density Polyethylene (LDPE), Polypropylene (PP), Polystyrene (PS), combustion time, pyrolytic oilAbstract
Abstract: This study aimed to determine which type of plastic – PET, HDPE, LDPE, PP, PS, and Others, produces most oil yield in terms of time of complete degradation, temperature and amount of plastics using a non-catalytic slow pyrolysis method; it also determined the physical characteristics of the oil yield in terms of its color and appearance; and it also aimed to determine which petroleum produced by different types of plastics are more efficient in terms of (a) production of oil and (b) combustion time. Production of oil and oil yield is presented in milliliters and percentage, respectively. Combustion time is expressed in seconds from the time of ignition to total disappearance of flame having 1ml of oil tested from each produced pyrolytic oil. Experimental-descriptive comparative method was used in determining the type of plastic that yields to most of pyrolytic oil. Based from gathered results, at constant temperature and amount of plastics, PS produced most petroleum at 29.5% oil yield followed by PP with 29%. While PET produced the least petroleum with 0.01% oil yield. Color varies at different types of plastics, given that PET and PP produces light brown color, LDPE produces light yellow while HDPE, PS and Others produces black color. PET, HDPE, PP, PS and Others produced liquefied petroleum while LDPE produces flammable wax product. PS produced most petroleum with 295ml (29.5%), and PET produced least oil with 1ml (0.01%). Combustion time varies at different types of plastics: PS at 145 seconds, PP at 141 seconds, HDPE at 115 seconds, Others at 78 seconds, LDPE at 77 seconds while PET produced non – flammable oil yield. Thus, PS is most efficient as an alternative fuel in terms of production and combustion time. For the betterment of similar study, future researchers are encouraged to test the pyrolytic oil yielded from different types of plastics in engine performance and machineries and the comparative performance to the available commercial fuels.
Downloads
Metrics
References
Lebreton, L., & Andrady, A. (2019). Future scenarios of global plastic waste generation and disposal. Palgrave Communications, 5(1). https://doi.org/10.1057/s41599-018-0212-7
Geyer, R., Jambeck, J. R., & Law, K. L. (2017). Production, use, and fate of all plastics ever made. Science Advances, 3(7). https://doi.org/10.1126/sciadv.1700782
Ryu, H. W., Kim, D. H., Jae, J., Lam, S. S., Park, E. D., & Park, Y. (2020). Recent advances in catalytic co-pyrolysis of biomass and plastic waste for the production of petroleum-like hydrocarbons. Bioresource Technology, 310, 123473. https://doi.org/10.1016/j.biortech.2020.123473
Hwang, Y., Farooq, A., Park, S. H., Kim, K. H., Lee, M., Choi, S. C., Kim, M. Y., Park, R., & Park, Y. (2019). NH3-induced removal of NOx from a flue gas stream by silent discharge ozone generation in a double reactor system. Korean Journal of Chemical Engineering, 36, 1291-1297. https://doi.org/10.1007/s11814-019-0325-8
Miandad, R., Barakat, M., Aburiazaiza, A. S., Rehan, M., Ismail, I., & Nizami, A. (2016). Effect of plastic waste types on pyrolysis liquid oil. International Biodeterioration & Biodegradation, 119, 239–252. https://doi.org/10.1016/j.ibiod.2016.09.017
Karta, I. W., Farmasari, S., & Ocampo, D. M. (2023). Online Assessment of Primary Students’ Cognitive, Psychomotor, and Affective Domains: Practices from Urban and Rural Primary Schools in Indonesia. In SHS Web of Conferences (Vol. 173, p.01014). EDP Sciences.https://eric.ed.gov/?id=ED629883
Erdogan, S. (2020). Recycling of waste plastics into pyrolytic fuels and their use in IC engines. Sustainable Mobility. https://doi.org/10.5772/intechopen.90639
Prurapark, R., Owjaraen, K., Saengphrom, B., Limthongtip, I., & Tongam, N. (2020). Effect of temperature on pyrolysis oil using High-Density polyethylene and polyethylene terephthalate sources from mobile pyrolysis plant. Frontiers in Energy Research, 8. https://doi.org/10.3389/fenrg.2020.541535
Heydariaraghi, M., Ghorbanian, S., Hallajisani, A., & Salehpour, A. (2016). Fuel properties of the oils produced from the pyrolysis of commonly-used polymers: Effect of fractionating column. Journal of Analytical and Applied Pyrolysis, 121, 307–317. https://doi.org/10.1016/j.jaap.2016.08.010
Singh, R., Ruj, B., Sadhukhan, A., & Gupta, P. (2019). Thermal degradation of waste plastics under non-sweeping atmosphere: Part 1: Effect of temperature, product optimization, and degradation mechanism. Journal of Environmental Management, 239, 395–406. https://doi.org/10.1016/j.jenvman.2019.03.067
Saeaung, K., Phusunti, N., Phetwarotai, W., Assabumrungrat, S., & Cheirsilp, B. (2021). Catalytic pyrolysis of petroleum-based and biodegradable plastic waste to obtain high-value chemicals. Waste Management, 127, 101–111. https://doi.org/10.1016/j.wasman.2021.04.024
Sogancioglu, M., Ahmetli, G., & Yel, E. (2017). A comparative study on waste plastics pyrolysis liquid products quantity and energy recovery potential. Energy Procedia, 118, 221–226. https://doi.or/10.1016/j.egypro.2017.07.020
Miandad, R., Rehan, M., Barakat, M. A., Aburiazaiza, A. S., Khan, H., Ismail, I. M. I., . . . Nizami, A. S. (2019). Catalytic pyrolysis of plastic waste: Moving toward pyrolysis based biorefineries. Frontiers in Energy Research, 7. https://doi.org/10.3389/fenrg.2019.00027
Sonawane, Y. & Shindikar, M., & Khaladkar, M. (2017). High calorific value fuel from household plastic waste by catalytic pyrolysis, nature environment and pollution technology. Nature Environment and Pollution Technology. 16(3). 879-882. Retrieved on 6 March 2021 from http://www.neptjournal.com/upload-images/NL-61-29-(27)B-3435.pdf
Paraschiv, M., Kuncser, R., Tazerout, M., & Prisecaru, T. (2015). New energy value chain through pyrolysis of hospital plastic waste. Applied Thermal Engineering, 87, 424–433. https://doi.org/10.1016/j.applthermaleng.2015.04.070
Lee, K. H. (2012). Effects of the types of zeolites on catalytic upgrading of pyrolysis wax oil. Journal of Analytical and Applied Pyrolysis, 94, 209–214. https://doi.org/10.1016/j.jaap.2011.12.015
FakhrHoseini, S. M., & Dastanian, M. (2013). Predicting pyrolysis products of PE, PP, and PET using NRTL activity coefficient model. Journal of Chemistry, 2013, 1–5. https://doi.org/10.1155/2013/487676
Abnisa, F., & Wan Daud, W. M. A. (2014). A review on co-pyrolysis of biomass: An optional technique to obtain a high-grade pyrolysis oil. Energy Conversion and Management, 87, 71–85. https://doi.org/10.1016/j.enconman.2014.07.007
Sharuddin, S. D., Abnisa, F., Wan Daud, W. M. A., & Aroua, M. K. (2016). A review on pyrolysis of plastic wastes. Energy Conversion and Management, 115, 308–326. https://doi.org/10.1016/j.enconman.2016.02.037
Zannikos, F., Kalligeros, S., Anastopoulos, G., & Lois, E. (2013). Converting biomass and waste plastic to solid fuel briquettes. Journal of Renewable Energy, 2013, 1–9. https://doi.org/10.1155/2013/360368
Odejobi, O., Oladunni, A., Sonibare, J., & Abegunrin, I. (2020). Oil yield optimization from co-pyrolysis of low-density polyethylene (LDPE), polystyrene (PS) and polyethylene terephthalate (PET) using simplex lattice mixture design. Fuel Communications, 2–5, 100006. https://doi.org/10.1016/j.jfueco.2020.100006
Akubo, K., Nahil, M. A., & Williams, P. T. (2017). Aromatic fuel oils are produced from the pyrolysis-catalysis of polyethylene plastic with metal-impregnated zeolite catalysts. Journal of the Energy Institute, 92(1), 195–202. https://doi.org/10.1016/j.joei.2017.10.009
Ahmad, I., Khan, M. I., Khan, H., Ishaq, M., Tariq, R., Gul, K., & Ahmad, W. (2014). Pyrolysis study of polypropylene and polyethylene into premium oil products. International Journal of Green Energy, 12(7), 663–671. https://doi.org/10.1080/15435075.2014.880146
Wu, J., Chen, T., Luo, X., Han, D., Wang, Z., & Wu, J. (2014). TG/FTIR analysis on co-pyrolysis behavior of PE, PVC and PS. Waste Management, 34(3), 676–682. https://doi.org/10.1016/j.wasman.2013.12.005
Siddiqui, M. N., & Redhwi, H. H. (2009). Pyrolysis of mixed plastics for the recovery of useful products. Fuel Processing Technology, 90(4), 545–552. https://doi.org/10.1016/j.fuproc.2009.01.003
Ding, C., Zhou, D. C., Zhang, X. H., Ma, X., & Wang, J. (2014). A relationship between flash point and boiling point of the flammable liquids at low pressure. Applied Mechanics and Materials, 664, 210–214. https://doi.org/10.4028/www.scientific.net/amm.664.210
Sharuddin, S. D. A., Abnisa, F., Daud, W. M. A. W., & Aroua, M. K. (2018). Pyrolysis of plastic waste for liquid fuel production as prospective energy resource. IOP Conference Series: Materials Science and Engineering, 334, 012001. https://doi.org/10.1088/1757-899x/334/1/012001
Kabakcı, S. B., & Hacıbektaşoğlu, E. (2017). Catalytic pyrolysis of biomass. Pyrolysis. https://doi.org/10.5772/67569
Stefanidis, S. D., Kalogiannis, K. G., Iliopoulou, E. F., Michailof, C. M., Pilavachi, P. A., & Lappas, A. A. (2014). A study of lignocellulosic biomass pyrolysis via the pyrolysis of cellulose, hemicellulose and lignin. Journal of Analytical and Applied Pyrolysis, 105, 143–150. https://doi.org/10.1016/j.jaap.2013.10.013
Collard, F. X., Blin, J., Bensakhria, A., & Valette, J. (2012). Influence of impregnated metal on the pyrolysis conversion of biomass constituents. Journal of Analytical and Applied Pyrolysis, 95, 213–226. https://doi.org/10.1016/j.jaap.2012.02.009
Aguado, J., Serrano, D., Escola, J., Garagorri, E., & Fernández, J. (2000). Catalytic conversion of polyolefins into fuels over zeolite beta. Polymer Degradation and Stability, 69(1), 11–16. https://doi.org/10.1016/s0141-3910(00)00023-9
Ma, C., Yu, J., Wang, B., Song, Z., Xiang, J., Hu, S., ... & Sun, L. (2017). Catalytic pyrolysis of flame retarded high impact polystyrene over various solid acid catalysts. Fuel Processing Technology, 155, 32-41.
Qian, K., Kumar, A., Zhang, H., Bellmer, D., & Huhnke, R. (2015). Recent advances in utilization of biochar. Renewable and Sustainable Energy Reviews, 42, 1055-1064.
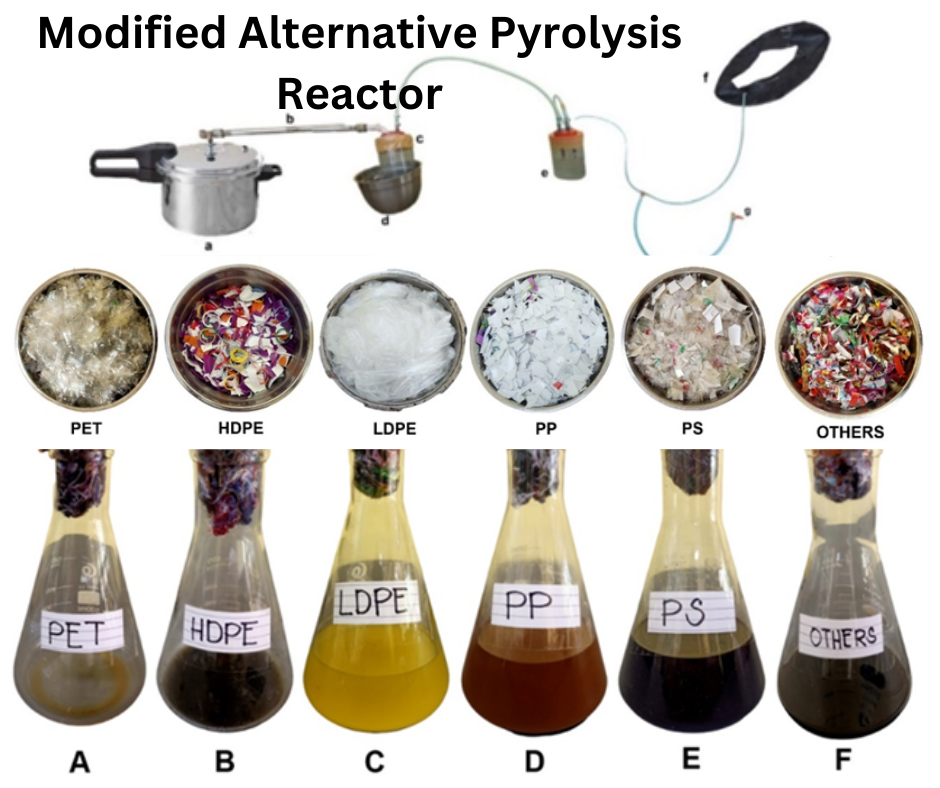
Downloads
Published
How to Cite
Issue
Section
License
Copyright (c) 2024 Nelson Jun Herrero, Rosenda Azañon, Abbie Jane Alvaro, Darrel Ocampo

This work is licensed under a Creative Commons Attribution-NonCommercial-ShareAlike 4.0 International License.
Authors who publish with ACA: Acta Chimica Asiana agree to the following terms:
- Authors retain copyright and grant the journal right of first publication with the work simultaneously licensed under a Creative Commons Attribution-NonCommercial-ShareAlike 4.0 International License. This license allows authors to use all articles, data sets, graphics, and appendices in data mining applications, search engines, web sites, blogs, and other platforms by providing an appropriate reference. The journal allows the author(s) to hold the copyright without restrictions and will retain publishing rights without restrictions.
- Authors are able to enter into separate, additional contractual arrangements for the non-exclusive distribution of the journal's published version of the work (e.g., post it to an institutional repository or publish it in a book), with an acknowledgement of its initial publication in ACA: Acta Chimica Asiana.
- Authors are permitted and encouraged to post their work online (e.g., in institutional repositories or on their website) prior to and during the submission process, as it can lead to productive exchanges, as well as earlier and greater citation of published work (See The Effect of Open Access).